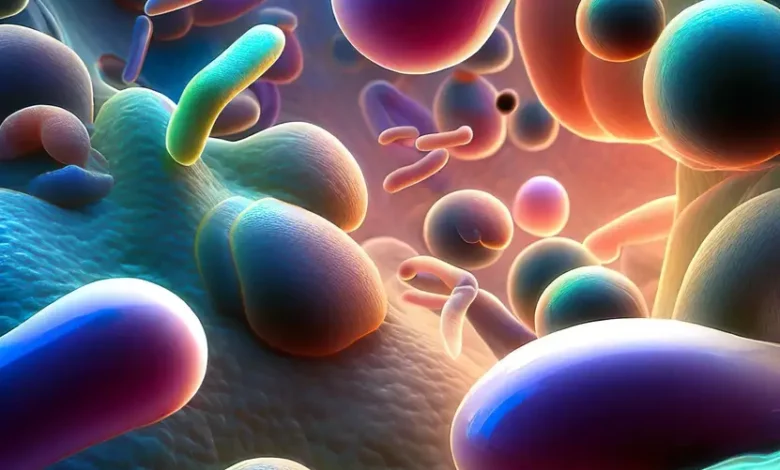
We have long known that biological materials absorb ambient moisture. But new research from Columbia shows that ambient water is much more central to the character of natural materials such as pine cones, fungi, and other plants and trees than was previously known.
A recent study argues that materials like wood, bacteria, and fungi belong to a newly identified class of matter, “hydration solids.”
For many years, the fields of physics and chemistry have held the belief that the properties of solid materials are fundamentally determined by the atoms and molecules they consist of. For instance, the crystalline nature of salt is credited to the ionic bond formed between sodium and chloride ions. Similarly, metals such as iron or copper owe their robustness to the metallic bonds between their respective atoms, and the elasticity of rubbers stems from the flexible bonds in the polymers that form them. This principle also applies to substances like fungi, bacteria, and wood.
Or so the story goes.
A new paper recently published in Nature upends that paradigm, and argues that the character of many biological materials is actually created by the water that permeates these materials. Water gives rise to a solid and goes on to define the properties of that solid, all the while maintaining its liquid characteristics.
In their paper, the authors group these and other materials into a new class of matter that they call “hydration solids,” which they say “acquire their structural rigidity, the defining characteristic of the solid state, from the fluid permeating their pores.” The new understanding of biological matter can help answer questions that have dogged scientists for years.
“I think this is a really special moment in science,” Ozgur Sahin, a professor of Biological Sciences and Physics and one of the paper’s authors, said. “It’s unifying something incredibly diverse and complex with a simple explanation. It’s a big surprise, an intellectual delight.”
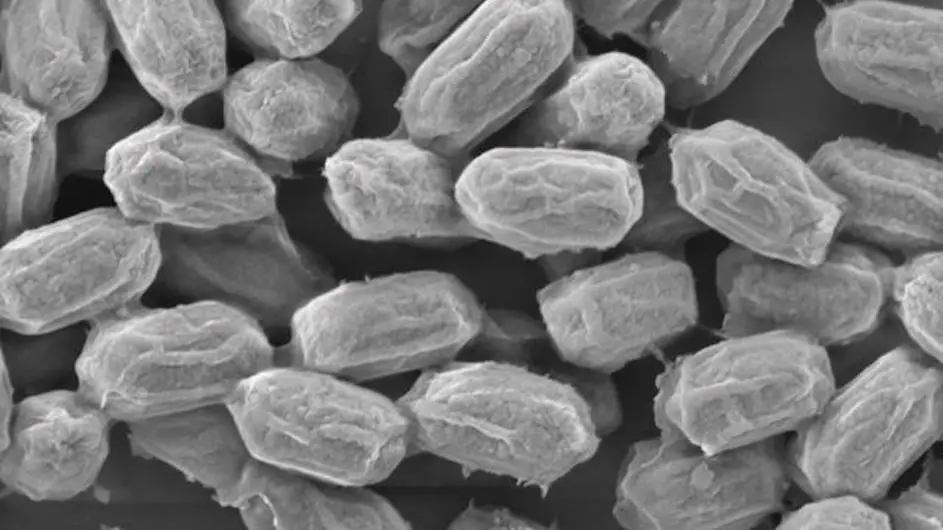
Steven G. Harrellson, who recently completed doctoral studies in Columbia’s physics department, and is an author on the study, used the metaphor of a building to describe the team’s finding: “If you think of biological materials like a skyscraper, the molecular building blocks are the steel frames that hold them up, and water in between the molecular building blocks is the air inside the steel frames. We discovered that some skyscrapers aren’t supported by their steel frames but by the air within those frames.”
“This idea may seem hard to believe, but it resolves mysteries and helps predict the existence of exciting phenomena in materials,” Sahin added.
When water is in its liquid form, its molecules strike a fine balance between order and disorder. But when the molecules that form biological materials combine with water, they tip the balance toward order: Water wants to return to its original state. As a result, the water molecules push the biological matter’s molecules away. That pushing force, called the hydration force, was identified in the 1970s, but its impact on biological matter was thought to be limited. This new paper’s argument that the hydration force is what defines the character of biological matter almost entirely, including how soft or hard it is, thus comes as a surprise.
We have long known that biological materials absorb ambient moisture. Think, for example, of a wooden door, that expands during a humid spell. This research, however, shows that ambient water is much more central to wood, fungi, plants, and other natural materials’ character than we had ever known.
The team found that bringing water to the front and center allowed them to describe the characteristics that familiar organic materials display with very simple math. Previous models of how water interacts with organic matter have required advanced computer simulations to predict the properties of the material. The simplicity of the formulas that the team found can predict these properties suggests that they’re onto something.
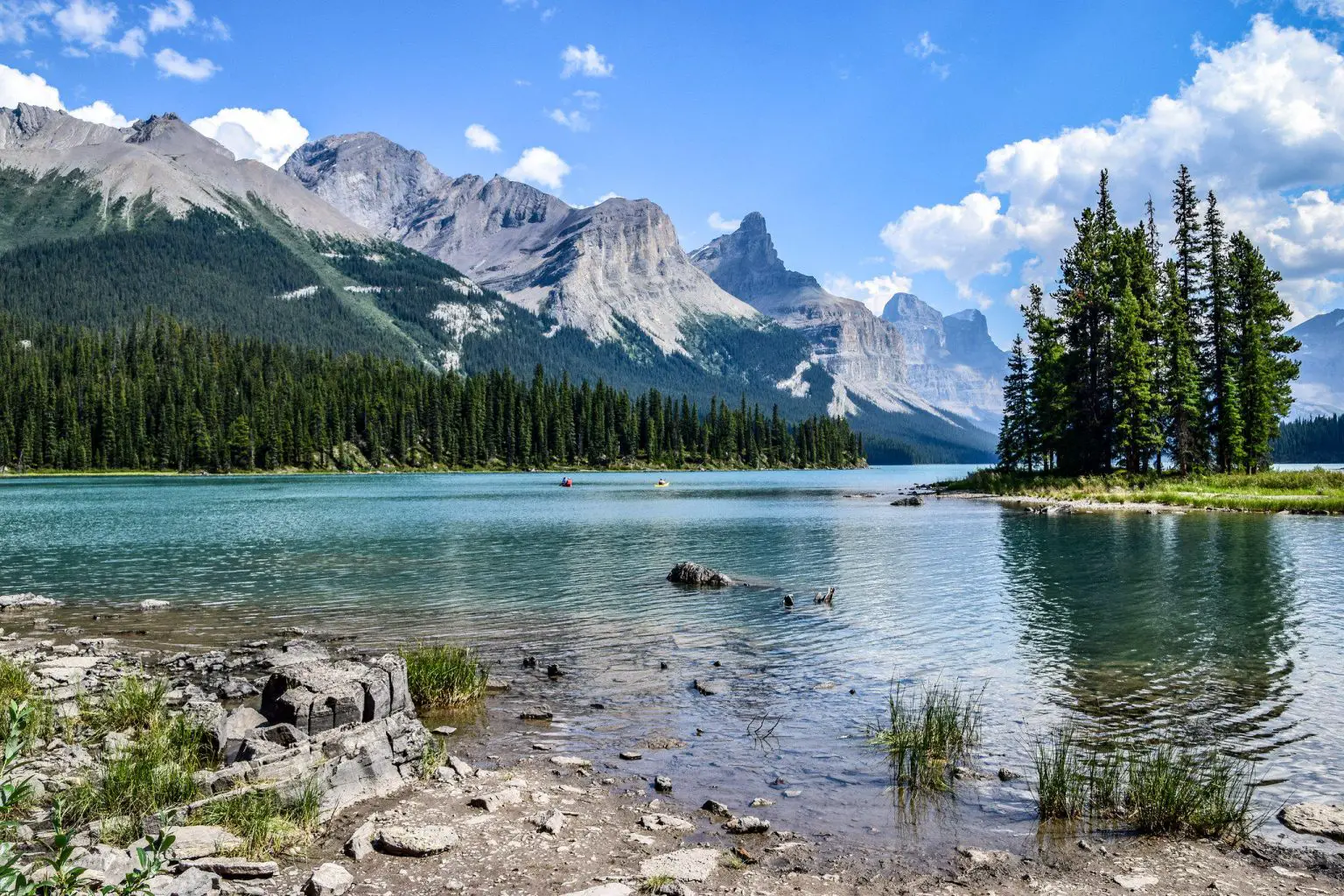
To take one example, the team found that the simple equation E=Al/λ neatly describes how a material’s elasticity changes based on factors including humidity, temperature, and molecule size. (E in this equation refers to the elasticity of a material; A is a factor that depends on the temperature and humidity of the environment; l is the approximate size of biological molecules and λ is the distance over which hydration forces lose their strength).
“The more we worked on this project, the simpler the answers became,” Harrellson said, adding that the experience “is very rare in science.”
The new findings emerged from Professor Sahin’s ongoing research into the strange behavior of spores, dormant bacterial cells. For years, Sahin and his students have studied spores to understand why they expand forcefully when water is added to them and contract when water is removed. (Several years ago, Sahin and colleagues garnered media coverage for harnessing that capability to create small engine-like contraptions powered by spores.)
Around 2012, Sahin decided to take a step back to ask why the spores behave the way they do. He was joined by researchers Michael S. DeLay and Xi Chen, authors on the new paper, who were then members of his lab. Their experiments did not provide a resolution to the mysterious behavior of spores. “We ended up with more mysteries than when we started,” Sahin remembers. They were stuck, but the mysteries they encountered were hinting that there was something worth pursuing.
After years of pondering potential explanations, it occurred to Sahin that the mysteries the team continually encountered could be explained if the hydration force governed the way that water moved in spores.
The team had to do more experiments to test the idea. In 2018, Harrellson, who is now a software engineer at the data analytics firm Palantir, joined the project.
“When we initially tackled the project, it seemed impossibly complicated. We were trying to explain several different effects, each with their own unsatisfying formula. Once we started using hydration forces, every one of the old formulas could be stripped away. When only hydration forces were left, it felt like our feet finally hit the ground. It was amazing, and a huge relief; things made sense,” he said.
The results of those experiments led the team and their collaborators to this paper. In addition to Harrellson, DeLay, Chen, and Sahin, the paper’s other authors are Ahmet-Hamdi Cavusoglu, Jonathan Dworkin, and Howard A. Stone. Adam Driks of Loyola University Chicago, who also contributed research, passed away before the completion of the work.
The paper’s findings apply to huge amounts of the world around us: Hygroscopic biological materials–that is, biological materials that allow water in and out of them–potentially make up anywhere from 50% to 90% of the living world around us, including all of the world’s wood, but also other familiar materials like bamboo, cotton, pine cones, wool, hair, fingernails, pollen grains in plants, the outer skin of animals, and bacterial and fungal spores that help these organisms survive and reproduce.
The term coined in the paper, “hydration solids,” applies to any natural material that’s responsive to the ambient humidity around it. With the equations that the team identified, they and other researchers can now predict materials’ mechanical properties from basic physics principles. So far that was true mainly of gases, thanks to the well-known general gas equation, which has been known to scientists since the 19th century.
“When we take a walk in the woods, we think of the trees and plants around us as typical solids. This research shows that we should really think of those trees and plants as towers of water holding sugars and proteins in place,” Sahin said: “It’s really water’s world.”
Reference: “Hydration solids” by Steven G. Harrellson, Michael S. DeLay, Xi Chen, Ahmet-Hamdi Cavusoglu, Jonathan Dworkin, Howard A. Stone and Ozgur Sahin, 7 June 2023, Nature.
DOI: 10.1038/s41586-023-06144-y
The study was funded by the U.S. Department of Energy, the Office of Naval Research, the National Institutes of Health, and the David and Lucile Packard Foundation.