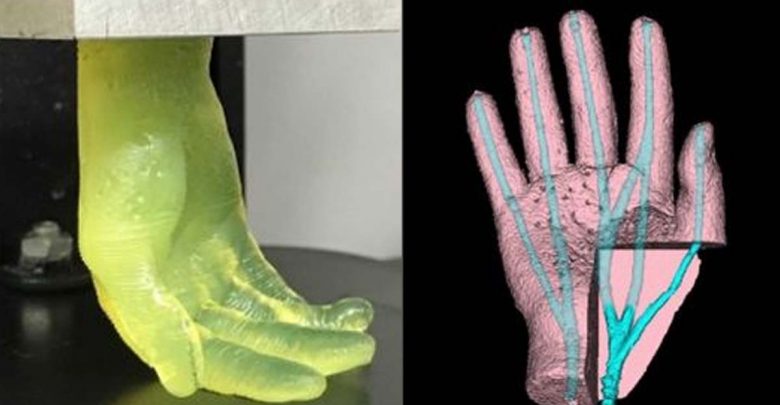
It looks like science fiction: A machine dips into a shallow vat of translucent yellow goo and pulls out what becomes a life-sized hand.
3D printing technologies have evolved at an unbelievable pace resulting in everything from 3D printed meat, to 3D printed houses to even 3D printed guns.
A new method in high-speed 3D printing utilizes stereolithography and hydrogels–jelly-like materials that speed up the process, making 3D printed organs possible in the near future.
Scientists from the University at Buffalo have developed a rapid new 3D bioprinting method that could represent a significant step towards fully-printed human organs.
Many 3D printers have boasted that they may be the future of printed organs but we haven’t gotten there just yet. Now, the study out of the University of Buffalo may just be the key to 3D printed organs.
Using a novel vat-SLA-based approach, the team have been able to reduce the time it takes to create cell-laden hydrogel structures, from over 6 hours to just 19 minutes. The expedited biofabrication method also enables the production of embedded blood vessel networks, potentially making it a significant step towards the lifesaving 3D printed organs needed by those on transplant waiting lists.
The rapid printing of centimeter-sized hydrogel models using FLOAT was shown to significantly reduce the part deformation and cellular injury caused by the prolonged exposure to the environmental stresses in layer-by-layer based printing methods. Media perfusion in the printed vessel network was shown to promote cell survival and metabolic function in the deep core of the large-sized hydrogel model over long term.
“Our method allows for the rapid printing of centimeter-sized hydrogel models,” explained the study’s lead co-author, Chi Zhou. “It significantly reduces part deformation and cellular injuries caused by the prolonged exposure to the environmental stresses you commonly see in conventional 3D printing.”
“The technology we’ve developed is 10-50 times faster than the industry standard, and it works with large sample sizes that have been very difficult to achieve previously.”
Taking bioprinting up a gear
Although bioprinted cell‐laden structures hold significant potential when it comes to human tissue and organ transplants, the technology is still at a nascent stage. One of the main hurdles facing the wider adoption of these processes is print speed, as the deposition rates of hydrogels have so far been limited to avoid damaging their incumbent cells.
Nozzle-based techniques have other drawbacks too, as they can cause prolonged cellular exposure to shear stress as well as low oxygen levels and temperatures, damaging them in the process. What’s more, the hydrogel scaffolds produced using conventional methods often exhibit low mechanical strength, making it difficult to incorporate soft overhanging structures like vascular channels.
The above is a 7 second video of a 19 minute 3D bioprinting in what becomes a life sized hand. Credit: University at Buffalo
While using sacrificial supports enables scientists to partially overcome this deficiency, the simplicity of the extrusion method behind this approach continues to limit its capacity. By contrast, recently-developed Continuous Liquid Interface Production (CLIP) technologies, have the potential to drastically increase the speed of bioprinting processes.
By continuously building layers above a ‘dead zone,’ CLIP methods allow materials to constantly be replenished, increasing production capacity, but at the cost of only being able to create thin-walled parts. Building on this approach, the Buffalo team have now developed a ‘FLOAT’ method, in which hydrogels can be deposited at a higher velocity, enabling the production of larger vascularized tissues.
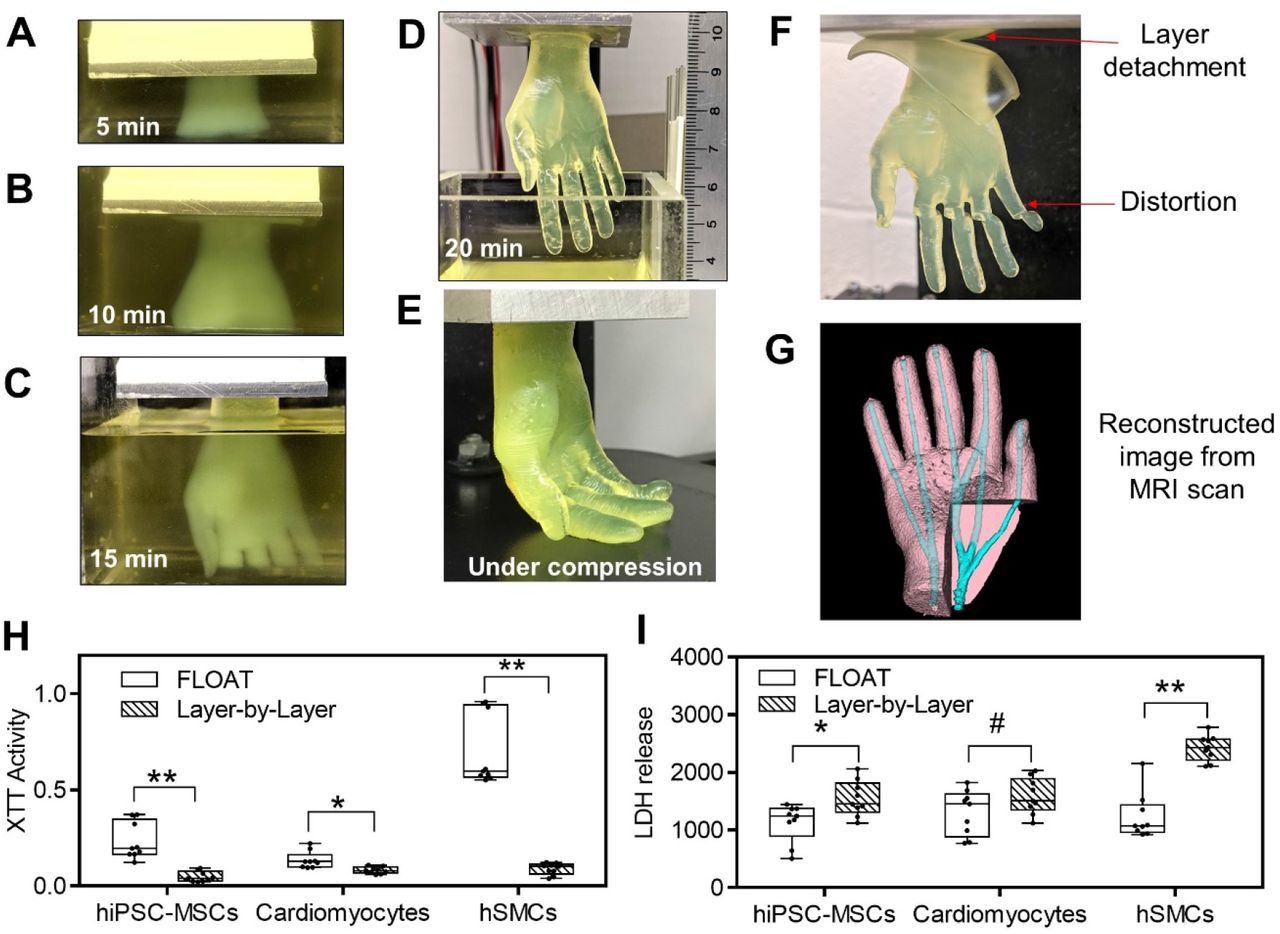
The ‘FLOAT’ bioprinting approach
During the researchers’ optimized FLOAT method, objects are essentially cured through a glass plate inside a vat of hydrogel at low suction forces, yielding thick parts with high elasticity. To prove the biocompatibility of their approach, the team initially fabricated a set of specimens from the cell-compatible PEGNB polymer.
Interestingly, while test parts exhibited sufficient rigidity, they also shrunk by up to 51%, causing the researchers to switch to a PEGDA material for larger models. In more ambitious test runs, the Buffalo team then 3D printed several 2.6 × 1.7 × 5.6 cm hand-shaped hydrogel structures, with ‘fingers’ that bent under compression.
Producing the same models using a normal SLA 3D printer took the team around 6.5 hours, significantly longer than the 19 minutes of their FLOAT-based machine. The scientists’ hydrogel-based hands also featured vascular channels, meaning that they could eventually be seeded with endothelial cells to create functional, transplantable limbs.
Ultimately, the scientists were able to seed patches of cells into microchannels ex-vivo, but they also found that integrating these into higher-strength structures yielded low cell viability. In future, the team believe that switching to nanomaterial-doped polymers could provide the answer to balancing rigidity and compatibility, and enable the rapid production of hydrogel-based vascularized structures.
Inching bioprinting towards reality
While the 3D bioprinting remains at a largely experimental stage, there are signs that the technology is slowly progressing towards more end-use applications.
3D printer OEM 3D Systems announced a major breakthrough in its Print to Perfusion bioprinting platform earlier this year. The system is now capable of creating fully-sized vascularized lung scaffolds, and the company has indicated that the technology will soon play a key role within its healthcare business.
Biotechnology firm United Therapeutics and Israeli company CollPlant have also made significant advances in their bid to mass-manufacture 3D printed kidneys. The companies have turned a former tobacco factory into a modern 3D bioprinting production line, which could be capable of churning out additive organs.
Elsewhere, efforts to create functional human organs have been limited to miniaturized models, such as the tiny 3D bioprinted hearts created by scientists at the University of Texas at El Paso. The vascularized structures were sent to the International Space Station (ISS) to test how microgravity affects the human heart.
The researchers’ findings are detailed in their paper titled “Fast Stereolithography Printing of Large‐Scale Biocompatible Hydrogel Models. ” The study was co-authored by Nanditha Anandakrishnan, Hang Ye, Zipeng Guo, Zhaowei Chen, Kyle I. Mentkowski, Jennifer K. Lang, Nika Rajabian, Stelios T. Andreadis, Zhen Ma, Joseph A. Spernyak, Jonathan F. Lovell, Depeng Wang, Jun Xia, Chi Zhou and Ruogang Zhao.