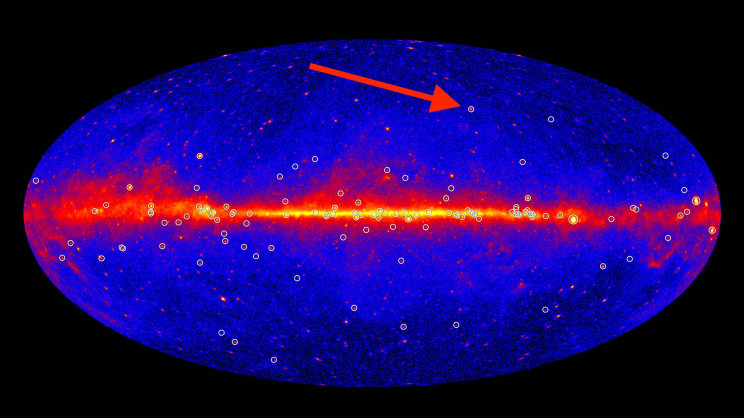
Our galaxy, the Milky Way, is on a collision course.
But don’t hold your breath.
In roughly 4.5 billion years, the Milky Way and its nearest galactic neighbor, Andromeda, will merge. These galaxies — like all the others — each have a supermassive black hole at their center. As gravity pulls them closer and closer together, the black holes will almost certainly begin orbiting each other.
These objects contain so much matter (the Milky Way’s black hole is roughly four million times more massive than the Sun) that their dance will send a spiraling ripple through the fabric of spacetime.
Cosmologists call those ripples “gravitational waves.” They can roll through the universe for a very, very long time.
In a study published Thursday in the academic journal Science, a team of researchers report how they used our galaxy as a gargantuan scientific instrument to detect ancient gravitational waves that have been rolling through the universe since the first galaxies began to merge billions and billions of years ago.
“These ripples in space-time all merge together… and ultimately produce this extremely long-wavelength background of gravitational waves,” cosmologist Aditya Parthasarathy, a co-author of the new study, told IE.
This is the first study to use gamma rays — electromagnetic radiation in its most energetic form — to take measurements of the gravitational wave background.
Our galaxy is full of dead stars that keep perfect time
If gravity had its way, the sheer mass of a star would cause it to collapse right away. And that’s exactly what happens to stars of a certain mass once they start creating atoms so heavy that fusing them together doesn’t create enough energy to push back against the patient, crushing pressure of gravity. As soon as it gets the upper hand, the star’s own gravity sends it into a dramatically violent collapse-explosion that produces either a small black hole or a very dense bundle of matter called a neutron star.
Neutron stars spin very fast, and some of them shoot out a chaotic beam of radiation that includes everything from low-energy radio waves to highly energetic gamma-ray photons.
Here’s why that’s important for studying billions-of-years-old gravitational waves: the beam doesn’t have to point along the neutron star’s axis of rotation. More often than not, it sweeps the sky like the spinning light on top of an old-fashioned fire truck. That means that Earth-bound astronomers observing from far away can only “see” the light when it’s pointed more or less straight at Earth. From that perspective, the object looks like a rapid pulse of light, which is why that kind of neutron star is called a pulsar.
“You can imagine a pulsar like a lighthouse out in the galaxy,” Parthasarathy says.
“Certain kinds of pulsars, called millisecond pulsars, rotate really, really fast and very, very precisely,” Parthasarathy says. “We can know, based on a very simple model of the pulsar’s rotation, exactly when to expect the pulse.”
These rapid flashes of light are an invaluable source of information for cosmologists, especially when they flash hundreds of times per second. Researchers have found more than 400 of these millisecond pulsars scattered across the Milky Way. Roughly one-third of them emit the gamma rays that were useful to Parthasarathy and his fellow researchers
“Most of those 130 [pulsars] aren’t bright enough to do this kind of analysis, so we get down to 30,” astrophysicist Matthew Kerr, another co-author, tells IE.
For almost 14 years, the Fermi Gamma-ray Space Telescope has orbited Earth at an altitude roughly a hundred miles higher than the International Space Station, quietly collecting evidence of gamma rays for researchers across the world.
“When the gamma-ray comes into our detector, we don’t focus it or anything like that. It hits an atom nucleus somewhere and basically explodes,” Kerr says. “It actually just makes a particle shower in our detector, and we recreate the original direction of the particle.”
“We realized a couple of years ago that if we were to go and look for these gravitational waves with our gamma-ray data, we’d actually be in the same ballpark as [the network of radio telescopes on Earth that monitor pulsars by detecting lower-frequency radio waves]. This was kind of a surprise to us because we hadn’t really thought about doing this before,” he says.
News of black hole collisions travels far
It’s time to zoom out. Way out. Nearly all the pulsars ever detected — and absolutely all of those that act as celestial beacons for astronomers — are located within our galaxy, the Milky Way. The pulses of light arrive at Earth after journeys of thousands of years. That’s because even light, which travels very fast, takes time to move across great distances.
But in the scheme of things, thousands of lightyears isn’t so far away, and thousands of years in the past isn’t so long ago.
Billions of years ago, before the Earth formed, entire galaxies were already swirling around. Every now and then, gravity would pull two of these galaxies toward each other.
“We know that at the heart of massive galaxies is a supermassive black hole,” Parthasarathy says. “In the early Universe, massive galaxies merged, which means that [their] supermassive black holes also merged.”
This is thought to have happened quite often over the course of cosmic history. But these are not straight-on collisions. Instead, the black holes orbit each other for some time before eventually falling into each other’s gravity. Probably. The details of how this endgame unfolds (cosmologists call it the “final parsec problem”) aren’t yet understood. Results from research like this could clear up the confusion.
Supermassive black holes are so massive— often containing something like hundreds of millions or billions of times the amount of matter in the Sun — that they orbit each other from a great distance. It can take a period of many years for a pair to make a full revolution around each other.
That amount of mass moving through space does something so difficult to imagine that it was literally Albert Einstein who, just over a century ago, first predicted that such a thing might happen.
“Each time a pair of supermassive black holes merge, they create ripples in spacetime,” Parthasarathy says.
Kerr describes the phenomenon as “changing the curvature” or “bending” spacetime.
Strictly speaking, it isn’t just supermassive black hole mergers that cause such a disturbance. “When matter accelerates, it generates gravitational waves,” Kerr says. Even a person waving their hand generates an infinitesimally small gravitational wave. The reason cosmologists focus on black hole mergers is that they are some of the few events that involve enough mass to create large enough ripples in spacetime for even the most cutting-edge technology to detect.
Cosmologists began detecting gravitational waves less than a decade ago
The first gravitational waves that astrophysicists detected came from a much smaller collision. It was just seven years ago when an observatory designed to monitor how long it takes photons to travel the length of a 2.5-mile-long tube (actually, two tubes, operating in unison), noticed the trip took slightly longer than normal. Since the speed of light is always the same, the change must have been to the distance those photons traveled. After a tremendous amount of confirmation, researchers concluded that the observatory, located in Louisiana and Washington state and collectively known as LIGO (Laser Interferometer Gravitational-Wave Observatory), must have detected a gravitational wave.
“LIGO sees black holes and neutron stars — things that are about the mass of our Sun — as they’re merging together,” Kerr says. “When they’re merging, they’re emitting copious amounts of gravitational waves [at a very high frequency].”
LIGO’s tubes are around 2.5 miles long because that’s the scale at which theorists expected such waves to be most easily measured.
“When LIGO searches for the stellar-mass black holes, the wavelength of the gravitational waves produced is of the order of a few tens to hundreds of kilometers between wave crests, which means that you just really need kilometer-long arms to detect those gravitational waves,” Parthasarathy says.
But the gravitational waves that supermassive black holes create are a different story.
“We’re looking for black holes that are hundreds of millions and billions of times the mass of the Sun,” Kerr says. “These waves are really low frequency… it takes years for one wave to go through one cycle.”
“The longer the gravitational waves you’re trying to search for, the bigger the detector you need,” Parthasarathy says.
This is where pulsars come in
“If you want to detect these things, you need detectors that are galaxy-sized. We don’t have the technology… to build detectors which are galaxy-sized,” Parthasarathy says.
“The cool thing is that you can use clocks, like pulsars spread across the galaxy, to actually do [the same kind of] experiment. You can think of your line of sight to each pulsar like a LIGO arm,” he says.
Just as the researchers at LIGO know exactly how long it should take photons to travel the length of the 2.5-mile arm, astronomers know exactly how long it should take the photons from each pulsar to travel from that point in the galaxy to the detector on Earth.
“When the gravitational wave background passes through one of the lines of sight [between a pulsar and Earth], the arrival time from that pulsar [will be] earlier or later by a billionth of a second,” Parthasarathy says.
“Because these gravitational waves are bending space-time itself and causing the light paths to go slightly differently, depending on exactly what gravitational wave is between us and them,” Kerr says. “We’re looking for these tiny little variations in the arrival times from pulsars.”
With the help of computers, the researchers can stitch together observations from the dozens of pulsars they’re monitoring to fill in the bigger picture.
“You can start seeing this pattern across multiple lines of sight… and when you detect such variation in arrival time between multiple pulsars, you believe that you have detected a gravitational wave background,” he says.
Gravitational hold contain incredible secrets
This new research sheds light on events that happened billions of years ago. How many galaxies were there? How were they distributed throughout the Universe? How did they grow and change over time? How do supermassive black holes behave when they come in contact with each other? How often do those events happen?
The gravitational waves these incredible events sent rippling out into space serve as records of our cosmic past that were completely unreadable until just a few years ago. They also offer hints at our cosmic future.