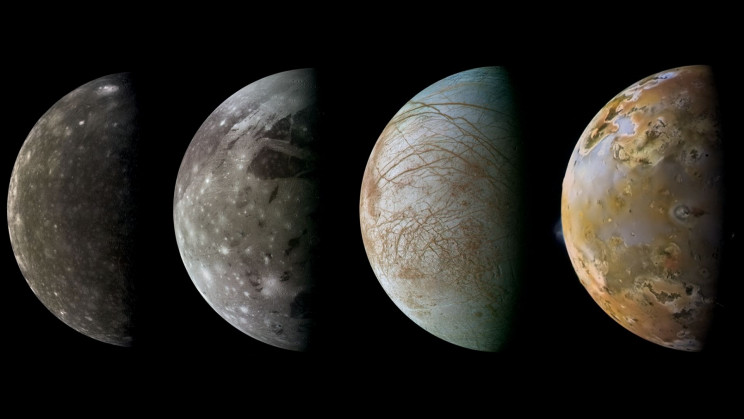
Lead Image: Jupiter’s Galilean Moons NASA’s Galileo spacecraft captured these images of Jupiter’s four largest moons. From left to right, they are shown in order from outermost to innermost: Callisto in 2001, Ganymede in 1997, Europa in 1998, and Io in 1997. Image Credit: NASA / JPL / Ted Stryk
What kind of challenges would humans face to live on Jupiter’s icy moons and what discoveries would await?
Today, we take a look at Jupiter’s largest moons. Also known as the “Galileans” or “Jovian Moons,” these satellites have long been a source of fascination for astronomers and scientists.
Ever since the Voyager 1 and 2 probes passed through the Jovian system in 1979, scientists have speculated that Europa may have an ocean beneath its icy surface. Since then, evidence has emerged that the same may be true for Ganymede, Callisto, and many other satellites in the Solar System.
In all cases, it is theorized that tidal flexing leads to hydrothermal activity at the core-mantle boundary. This is similar to what happens on Earth, where geological activity causes hydrothermal vents to form on the ocean floor. Since scientists believe that these vents may be where Earth’s earliest life forms arose, many also speculate that these “Ocean Worlds” (as they’re sometimes called) could support life.
As a result, multiple space agencies hope to send missions to the system in the near future to investigate these moons and search for possible signs of life. These include NASA’s Europa Clipper mission and the ESA’s Jupiter Icy Explorer (JUICE) missions, which will arrive around Jupiter in the 2030s.
There are even proposals for creating permanent settlements on Jupiter’s largest moons, especially Europa, Ganymede, and Callisto. These would give humans access to the Jovian System and its vast resources and serve as a stepping stone to Saturn and the outer Solar System.
The challenges associated with this are monumental, one might even say “herculean.” Luckily, the Jovian System contains considerable resources, tremendous opportunities for innovation, and immense benefits. With the right technology and a lot of effort, it may even be possible to establish settlements on Jupiter’s moons.
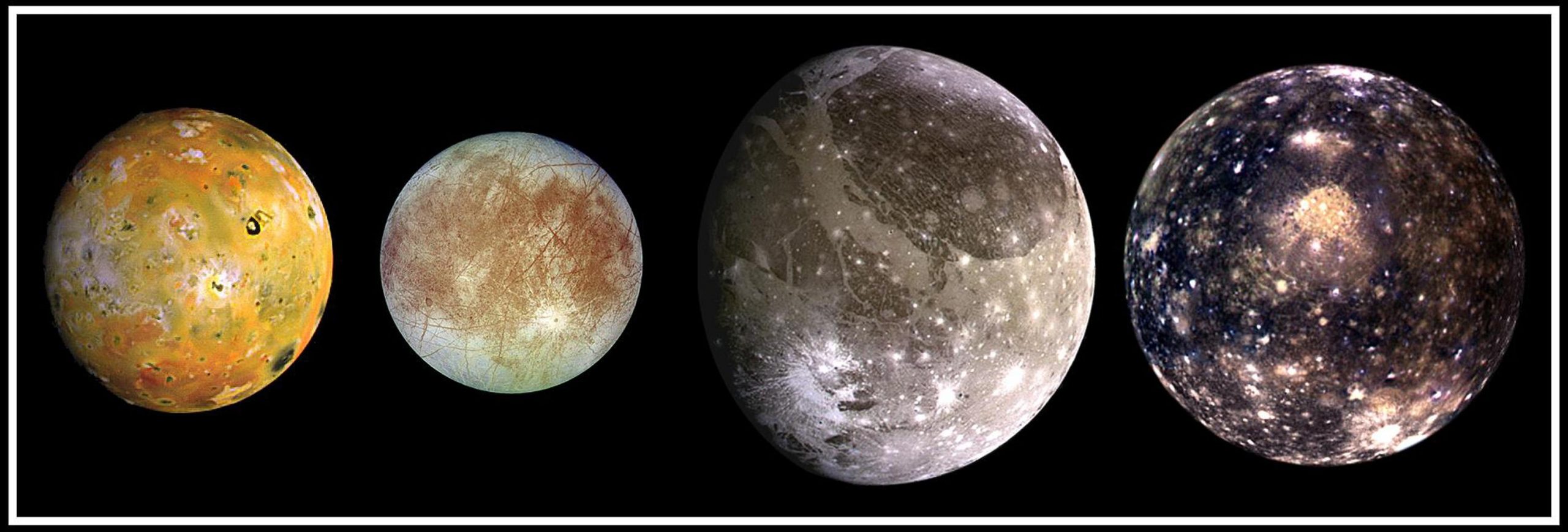
Jupiter’s moon ‘Ganymede’ is the largest and most massive moon in our solar system. It is approximately 26% larger than the planet Mercury by volume. This satellite of Jupiter is the ninth largest object of our solar system, after the sun and seven planets.
The four Galilean moons and four inner moons (Metis, Adrastea, Amalthea, and Thebe) of Jupiter are called regular satellites. These eight moons have prograde motion and orbit relatively near to Jupiter. Whereas the other moons of Jupiter are called irregular satellites. These irregular satellites of Jupiter have high eccentricity with their prograde and retrograde motion and have much inclination to Jupiter’s plane.
Some day, interplanetary tourists could be listening to messages like this:
“Welcome to the Jovian System! We hope that you enjoyed your time aboard Dyauspitar Station and have fully adapted to the local gravity. If you experience any symptoms of ‘gravity sickness,’ just use the Jovis app, which is free to download to your personal devices. Just tap the icon, and a medical team will be there in a flash to assist you. “
“Those heading to Callisto are invited to take a tour of the Great Craters! Our surface crawlers will whisk you to the dark side of the moon, where you can look upon the domes of Adlinda, Asgard, Utgard, and Valhalla! The city lights are beautiful. But even more impressive is Jupiter’s Great Red Spot, which will be making an appearance!”
“Those destined for Europa are invited to take a chartered tour of the Minoan, Rhadamanthean, and Aeacean Seas. Located within the ice sheet, these stations offer viewing opportunities for Europan sharks, rays, and other indigenous lifeforms. We remind all of our guests that private tours, especially to the deep ocean, are strictly forbidden.”
“Those bound for Ganymede are in for a real treat! Head on over to Enki Catena for a slow tram ride and witness the Galilean aurorae firsthand! Our crawlers are equipped with the most luxurious accommodations, which include King-sized viewing beds and crystal roof portals. Lay back and snuggle as you enjoy the clearest and most unobstructed view of the Galilean Lights!”
“We remind all guests that planetary protections are in place to ensure that the local biosphere is not contaminated. Bio scans are mandatory at all ports of call and the transfer of flora or fauna between habitats is punishable by law. And be sure to check your dosemeter regularly.”
The Galileans
Jupiter’s largest moons – Io, Europa, Ganymede, and Callisto – are collectively referred to as the “Galileans” in honor of Galileo Galilee (who discovered them in 1610 using a telescope of his own design). While Galileo initially tried to name them in honor of his patrons, the House of Medici in Florence, they ended up taking their names from Greek mythology after lovers of Zeus (Jupiter in the Roman pantheon).
Unlike Jupiter’s many other satellites, these were the only four massive enough to achieve hydrostatic equilibrium (become spherical). They are largely composed of ice, volatiles, silicate minerals, and metals – differentiated between a crust, a liquid mantle, and a core of rock and metal. Their densities and the level of radiation they are exposed to decrease with the distance of their orbit.
The innermost of these moons, Io measures 2263 miles in diameter (slightly larger than the Moon) and is named after the priestess of Hera in Greek mythology. Unlike most satellites in the outer Solar System, Io is primarily composed of silicate rock and metal and has the highest density of any known satellite.
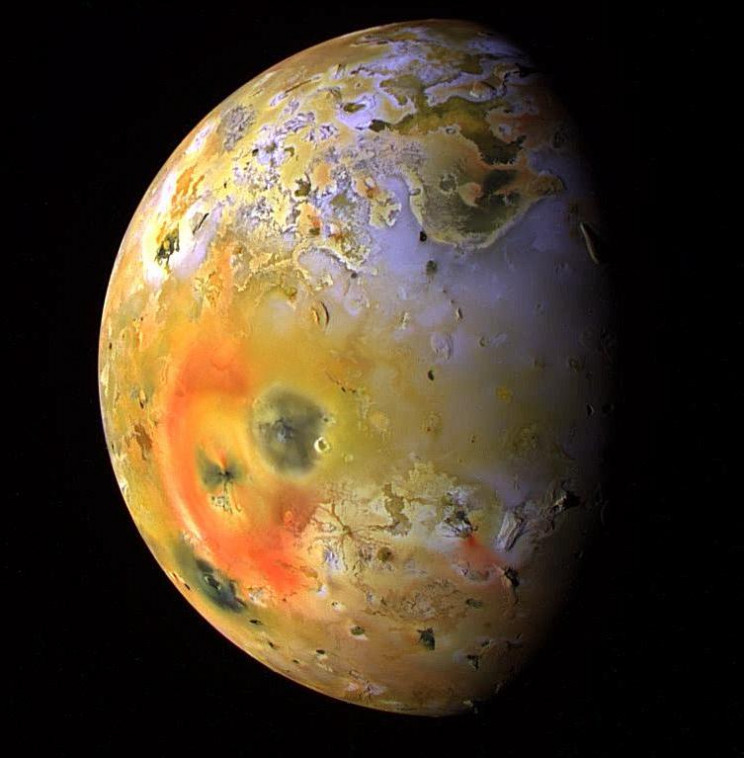
Models of Io’s interior indicate that it is differentiated between a silicate-rich crust and mantle, a molten interior, and a solid core made rich in iron or iron-sulfide. Io has the least amount of water of any known body in the Solar System, though small pockets of water ice or hydrated minerals have been tentatively identified.
Io is one of the most geologically active objects in the Solar System, with over 400 active volcanoes and more than 100 mountains (some taller than Mount Everest), and lava plumes that have been seen reaching up to 300 miles into space. Io has a very thin atmosphere made up of volcanic gases like sulfur dioxide (SO2), sulfur monoxide (SO), sodium chloride (NaCl), sulfur, and oxygen.
Io experiences aurorae as a result of charged particles from Jupiter’s magnetosphere interacting with its atmosphere. These aurorae are brightest near Io’s equator due to the lack of an intrinsic magnetic field, which causes electrons traveling from Jupiter to impact its atmosphere directly.
Next up is Europa, which was named after a Phoenician noblewoman and the daughter of the king of Tyre, who later became a lover of Zeus and the queen of Crete. With a diameter of about 1,940 miles and a mass of 10.58 ×1022 lbs, Europa is slightly smaller than Earth’s Moon and 65% as massive.
Europa is significantly less dense than any of the other Galilean Moons, which indicates that its composition is similar to most moons in the outer Solar System – i.e., differentiated between a rock interior composed of silicate rock and a possible iron core. Above this rocky interior is a layer of water ice that is estimated to be around 62 miles thick.
This layer is likely differentiated between a frozen upper crust and a warmer liquid ocean beneath. If present, this ocean is likely salty, contains organic molecules, and maybe oxygenated and heated by Europa’s geologically-active core. The presence of this liquid water interior and geological activity is bolstered by evidence of cryovolcanic activity surface plumes.
The prominent markings crisscrossing Europa (called lineae) are another major feature. These are believed to be warmer ice layers that were exposed to the surface through a series of eruptions of geological activity. This is similar to what takes place in Earth’s oceanic ridges, where convection forces material from the interior to migrate to the surface and vice versa.
Europa has a tenuous atmosphere composed mainly of molecular oxygen (O2), water vapor, and trace amounts of sodium. This atmosphere is the product of radiolysis, where UV radiation from the Sun and charged particles from Jupiter’s magnetosphere cause ice to sublimate and become chemically disassociated with hydrogen and oxygen gas.
The hydrogen, being very light, is largely lost to space while the oxygen is largely retained. Because the surface may interact with the subsurface ocean, this molecular oxygen may make its way to the ocean, where it could aid in biological processes.
This “neutral cloud” has been detected by both the Cassini and Galileo spacecraft and has a greater content (number of atoms and molecules) than the neutral cloud surrounding Jupiter’s inner moon Io. Models predict that almost every atom or molecule in Europa’s torus is eventually ionized, thus providing a potential source for Jupiter’s magnetospheric plasma.
Next up is Ganymede, named after the young boy who was carried to Olympus by Zeus (disguised as an eagle) and became the cupbearer of the Olympian gods. With a diameter of about 3275 miles, Ganymede is the largest moon in the Solar System, even larger than the planet Mercury (though only half as massive), and is the only satellite known to have a magnetic field.
Based on its size and density, Ganymede’s is believed to be composed of equal parts water ice and silicate rock. Its structure is fully-differentiated between a solid iron inner core, a liquid iron/iron-sulfide outer core, a silicate mantle, and a spherical outer icy shell. The most compelling evidence for the existence of a liquid, iron-nickel-rich core is Ganymede’s intrinsic magnetic field.
Scientists also believe that Ganymede has a deep ocean nestled between two layers of ice – a layer between the ocean core and a surface layer above it. The presence of this ocean has been confirmed by readings taken by orbiters and through studies of how Ganymede’s aurora behaves. These indicate that Ganymede’s auroras are affected by the moon’s magnetic field, which in turn is affected by the presence of a large, subsurface salt-water ocean.
Similar to Europa, Ganymede’s surface is rather smooth and consists of features that indicate geological activity and regular exchanges between the interior and the surface. For example, it has grooved terrain that is attributed to cryovolcanism and tidal flexing that heated the interior and strained the surface ice, leading to cracks, graben, and faults that led to its brighter and darker surface patches.
The outermost moon, Callisto, is named for the nymph who was associated with Artemis, the goddess of the hunt in the Greek pantheon. With a diameter of around 3,000 miles, Callisto is the third largest satellite in the Solar System and about 99% the size of Mercury. But due to its mixed composition, it has less than one-third the mass of Mercury.
Unlike the other Galileans, Callisto’s distant orbit means that it has never experienced much in the way of tidal heating, which has had a profound impact on its internal structure and evolution. Its average density suggests a composition of about equal parts of rocky material and water ice, with some additional volatile ices such as ammonia.
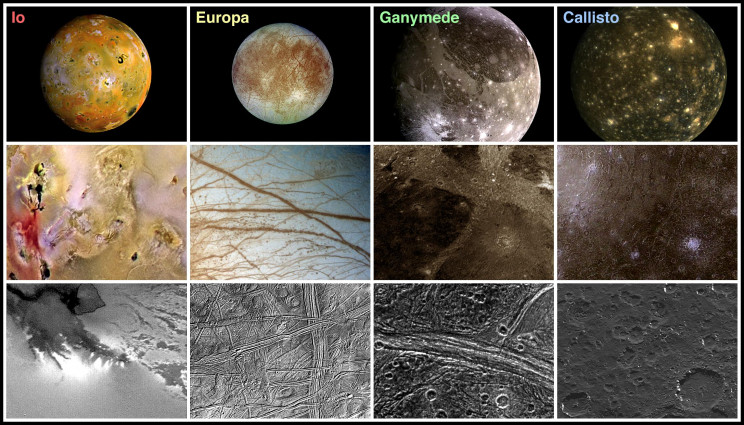
These are believed to be partially differentiated between an icy lithosphere, a salty ocean, an interior of compressed rocks and ices, and a small silicate core. Spectra obtained from the surface indicates that it consists of small, bright patches of pure water ice, intermixed with patches of a rock–ice mixture, and extended dark areas made of a non-ice material.
This consists of magnesium and iron-bearing hydrated silicates, carbon dioxide, sulfur dioxide, possibly ammonia, and various organic compounds. The presence of ammonia (an anti-freeze) and radioactive elements means that Callisto could have an interior ocean. Additional evidence for this ocean includes Jupiter’s magnetic field, which shows no signs of penetrating Callisto’s surface.
Its distance from Jupiter also means that the charged particles from Jupiter’s magnetosphere have had a very minor influence on its surface. Compared to the other Galileans, Callisto’s surface is saturated by ancient craters, with newer ones formed over older ones. What’s more, impact craters and their associated structures are the only large features on the surface.
The largest impact features on Callisto’s surface are multi-ring basins, which probably originated from post-impact concentric fracturing. The largest of these are Valhalla and Asgard, whose central, bright regions measure respectively 370 and 1000 miles in diameter and have rings that extend farther outwards.
Abundant resources
In his book Entering Space, Robert Zubrin described how the outer Solar System might one day become the “Persian Gulf” of the Solar System. In particular, Zubrin refers to the abundance of deuterium (H2) and Helium-3 (He3) in the atmospheres of gas giants like Jupiter and Saturn. These could be used as fuel in advanced fusion engines.
Jupiter’s magnetic field and atmospheric turbulence would make it difficult to harvest Helium-3 directly from its atmosphere. However, platforms built in orbit around the planet could facilitate operations where hardened robotic spacecraft (“skydivers” perhaps?) swoop into the atmosphere to scoop up hydrogen and helium.
These platforms could be equipped with facilities to manufacture the spacecraft and service them between runs. They could also serve as refining and refueling stations, where the gas would be turned into H2 and He3 fuel and made available for spacecraft passing to and from the Jovian System, or headed deeper into the Solar System.
The Jovian system also includes countless smaller moons and asteroids that are rich in metals, volatiles (water, carbon dioxide, ammonia), silicate minerals, and organic molecules. Asteroids that share Jupiter’s orbit are known as Trojans, which are differentiated between the “Greek” camp (those in the Sun-Jupiter L4 point and named for Greek heroes of the Trojan War) and the “Trojan” camp (those in the trailing L5 point and named for Trojan heroes).
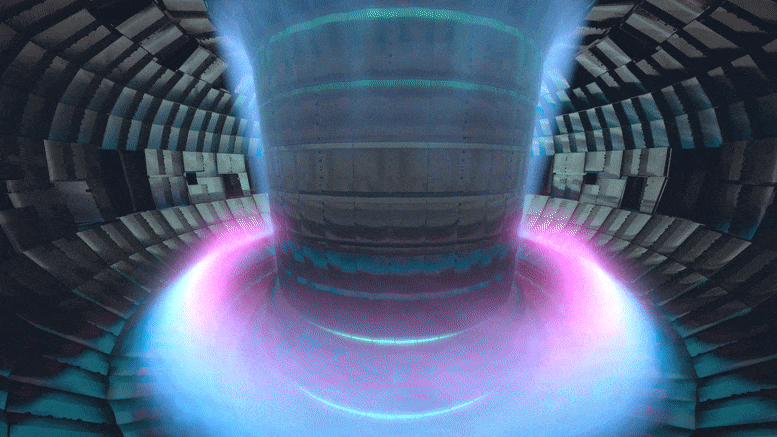
Based on their spectroscopy, the majority of Jupiter’s Trojans appear to be D-type asteroids, similar to what is found in the outer regions of the Main Asteroid Belt. These asteroids are theorized to be composed of an outer layer of organic-rich silicates, anhydrous silicates, and carbon that surround a water ice interior.
A smaller number are classified as C-type or P-type asteroids, the latter of which is thought to be similar in composition to D-type, but are rarer. C-type asteroids are the most common type in the Solar System and are composed of hydrogen, helium, hydrated minerals, and other volatiles.
All told, there are 80 satellites in orbit of Jupiter, which are divided between the regular and irregular groups. The regular group is subdivided into the inner satellites (aka. Amalthea Group) and the main group (Galileans). The former orbit very closely to Jupiter, have very short orbital periods and are believed to be similar in composition to the Galileans and Trojans (abundant water ice, volatiles, silicate minerals, and metals).
The irregular satellites are substantially smaller objects with more distant and eccentric orbits and are subdivided into those with prograde and retrograde orbits. These satellites are believed to be partially collision families that were created when larger parent bodies were shattered by asteroids captured by Jupiter’s gravitational field. Based on their spectra, these satellites appear to be a mix of C-type, S-type, and D-type bodies.
The abundance of water ice, especially in Europa, Ganymede, and Callisto, could also be used by the local settlers for everything from drinking and irrigation to the manufacture of oxygen and propellant. This could also facilitate the construction of fuel depots in the Jovian system that would be used by spacecraft coming to and from the outer Solar System.
Challenges & hazards
But of course, establishing a human presence around Jupiter also comes with its share of dangers, and they are pretty monumental!
The most obvious challenge when it comes to establishing human outposts in the Jovian system is the time it would take to make even a one-way trip. Over time, Jupiter and Earth vary considerably in their distance from each other, going from 365 million miles apart at their closest to 601 million miles at their farthest.
This situation is similar to traveling between Earth and Mars, where launch windows occur only every 26 months when the two planets are at the closest points in their orbit to each other (known as an “Opposition”). During these windows, a one-way mission will still take six to nine months to get there. In Jupiter’s case, launch windows are a bit more common, occurring every 398.9 days – roughly a year and a month.
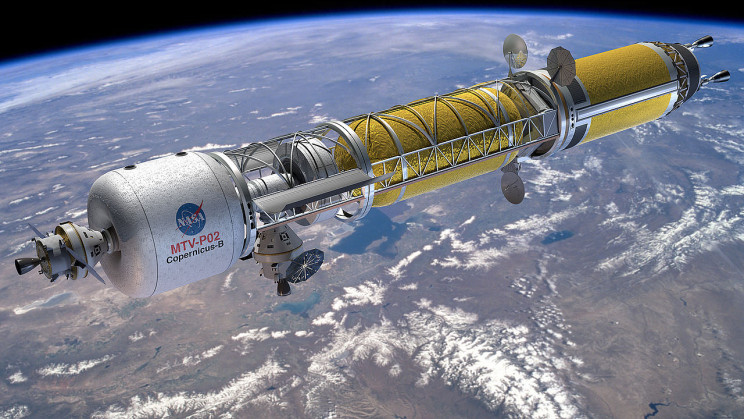
Image Credit: NASA
However, getting to Jupiter using current propulsion technology would take ten times as long – between 5 and 7.5 years! Once again, Zubrin acknowledged this challenge in his book, Entering Space:
“For while the ballistic interplanetary trajectories are made possible by chemical or nuclear thermal propulsion are adequate for human exploration of the inner Solar System and unmanned probes beyond, something a lot faster is going to be needed to sustain interplanetary commerce encompassing the gas giants.”
The idea of nuclear rockets was first proposed during the Space Race and research efforts bore fruit in the form of the “Nuclear Engine for Rocket Vehicle Application” (NERVA) concept. At present, NASA is revitalizing the idea in the form of nuclear-electric and nuclear-thermal propulsion (NEP/NTP) applications.
However, NASA estimates that such a propulsion system could reduce transit times between Earth and Mars to 100 days. For Jupiter, a single transit would take 1000 days (2.74 years), which is still a very long time for crews to remain in space (and that is just one way – they would still need to get home). Spacecraft destined for Jupiter would therefore need plenty of supplies, bioregenerative life support systems, advanced waste recycling/disposal methods, and methods for mitigating the hazards of radiation and microgravity.
Of the Galilean Moons, the outermost three are known to have tentative atmospheres primarily composed of molecular oxygen (O2), some water vapor, and traces of sodium. As noted, this is the result of solar UV radiation and charged particles from Jupiter’s magnetosphere interacting with surface ice to create water vapor, which is then broken down into hydrogen and oxygen.
This process is known as radiolysis and causes the hydrogen, being very light, to be lost to space while the heavier molecular oxygen is retained. Callisto also shows signs of having trace amounts of carbon dioxide (CO2) in its atmosphere while Io has a very thin atmosphere made up of volcanic gases – SO2, SO, NaCl, S, and O.
Unfortunately, none of these atmospheres are dense enough to retain heat or be breathable by Earth lifeforms. Based on multiple observations, the surface pressure of the Galilean Moons’ atmospheres is estimated to be less than one nano pascal (μPa) – one-trillionth of Earth’s atmospheric pressure (101,325 Pa).
Another major hazard is the intense radiation the Galilean moons are exposed to. In addition to the usual threats posed by cosmic and solar radiation, there’s Jupiter’s intense magnetic field, which bombards the moons with charged particles. For astronauts and settlers, long-term exposure to this radiation could lead to serious health problems.
As the innermost moon, Io is buried deep within Jupiter’s magnetic field and is exposed to an average of 36 sieverts (Sv) a day (or 13,140 Sv/year). The situation is better for Europa, which gets 5.4 sieverts a day (1,971 Sv/year); while Ganymede and Callisto are exposed to an average of 0.08 and 0.0001 Sv/day (29.2 and 0.0365 Sv/year).
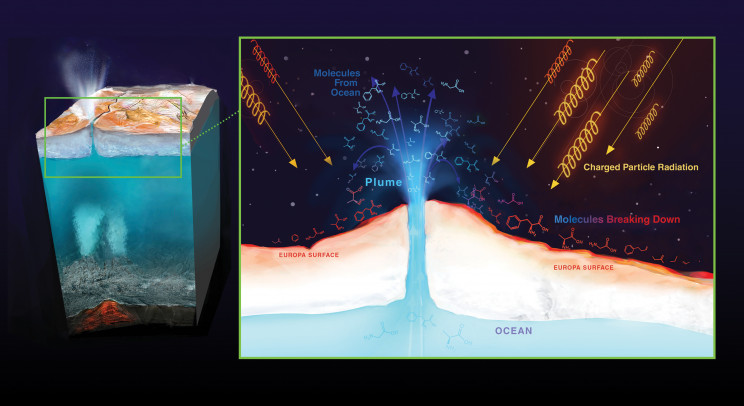
Credits: NASA/JPL-Caltech
According to the National Council on Radiation Protection and Measurements (NCRP) people living in developed nations are exposed to an average of 6.2 millisieverts (620 millirems) per year. To ensure their health and safety, NASA has established an upper limit of 50 mSv/year (50 rem/year) for astronauts operating aboard the International Space Station (ISS) and other missions in Low Earth Orbit (LEO).
This means that of the Galilean moons, only Callisto meets the radiation requirement for astronaut health and safety, with Ganymede ranking as a distant second. Unfortunately, as Robert Zubrin noted in Entering Space, radiation from cosmic sources and Jupiter’s magnetotail still pose a threat:
“There sill would be the normal cosmic-ray doses of about 0.14 rem/day. Also, while doses from Jupiter’s belts would be negligible under normal circumstances, they would be much higher when the satellites occasionally pass through Jupiter’s enormous magnetotail, which extends in the anti-sunward direction from Jupiter for hundreds of millions of kilometers. Presumably, however, people could take cover underground during these occurrences.”
This same strategy could be used for Europa, but in far stricter ways. On this moon, habitats will have to take advantage of the surface ice for protection. Beyond that, strategies will need to be developed to ensure the health and safety of anyone living on (or within) the surface ice.
Another major issue for the Jovian system is low gravity. On the Galilean moons, the surface gravity ranges from a low of 12.6% Earth-normal (0.126 g) for Callisto to 18.3% (0.183 g) for Io. Long-term exposure to low gravity is likely to lead to muscle atrophy, bone density loss, diminished organ function, and psychological and cognitive issues as well.
Well… that’s quite the list of challenges and hazards, isn’t it? But with the right technology and strategies, humans could actually make a life for themselves in the Jovian system.
Solutions for living
Since the Voyager probes passed through the Jovian system, several proposals have been made for crewed missions to Jupiter’s moons and even the creation of settlements. Each of these studies addressed the aforementioned challenges and proposed some common solutions. For instance, getting to and from the Jovian system will require more advanced propulsion and life support systems.
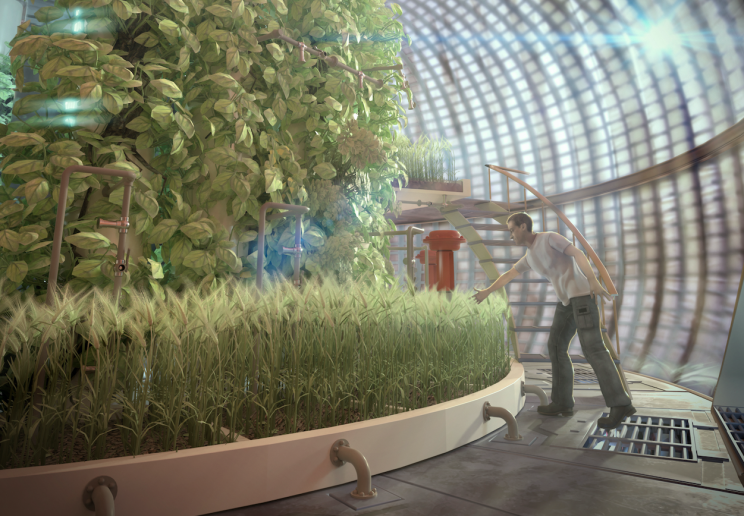
A joint 2003 study by NASA and the Ohio Aerospace Institute (OAI), titled “Revolutionary Concepts for Human Outer Planet Exploration” (HOPE) proposed a 5-year crewed mission to Callisto starting in the 2040s. The spacecraft that would transport the crew would be equipped with Nuclear-Electric Propulsion (NEP) and a rotating section to simulate gravity.
Nuclear-Thermal Propulsion (NTP) is another concept that NASA is considering for the sake of deep-space missions. As noted, these have the potential or reducing transit times to 1000 days, which is still considerable. However, this could be doable with the right kind of bioregenerative life support systems.
In particular, a rotating space module similar to NASA’s Non-Atmospheric Universal Transport Intended for Lengthy United States Exploration, Nautilus-X, the concept could combat the effects of microgravity in transit. Upon arrival, it could be repurposed as a space station (temporary or permanent) that settlers could use to get a little “gravity therapy.”
A report by the non-profit Lifeboat Foundation indicated that the Jovian moons are preferable destinations beyond the Moon and Mars because their surface gravity is comparable to the Moon (0.165 g). The creation of larger pinwheel stations throughout the Jovian system that would simulate 1 g would provide a long-term solution to the effects of low gravity.
Meanwhile, habitats could be built in Callisto’s many craters, which could be enclosed in large domes. Within these domes, entire ecosystems could be engineered by placing a layer of powdered regolith (harvested from nearby rocky bodies) on top of the surface ice, then adding organic molecules and water to create soil.
Over time, plants and animals could be imported to create a self-sustaining ecosystem that would replenish the water and air and provide regular sources of food. On Europa and Ganymede, multiple studies have shown that habitats could be built within “air pockets” in the ice sheet to provide natural shielding against radiation.
According to Rich Terrile, the deputy project scientist at NASA’s Jet Propulsion Laboratory, 6 feet (2 meters) of ice on Europa would be adequate for this. NASA JPL astrobiologist Steve Vance further indicates that the leading side of Europa (which faces away from Jupiter) is the best location for a base since exposure to radiation there is lower.
While a settlement on Io would not be advisable, the moon’s richness in terms of molten metals and volcanoes could constitute a tremendous power source for the Jovian System. Meanwhile, Europa, Ganymede, and Callisto are rich in water volatile elements, and organic molecules. These could be used to create everything from oxygen and drinking water to fertilizers and propellants.
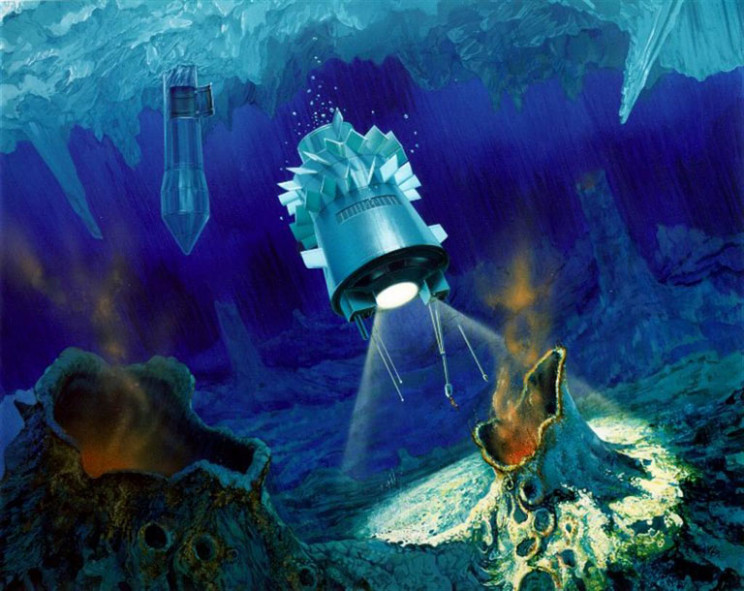
Jupiter’s powerful gravity and magnetic field could also be used as a power source. Io, Europa, and Ganymede all experience tidal flexing in their interior, a process that leads to hydrothermal activity at the core-mantle boundary. On Earth, this activity is the reason for hydrothermal events on the ocean floor.
Turbines placed around these vents would be able to use the moving water to generate electricity and possibly absorb the heat and chemical energy too. A more adventurous possibility would be to position piezoelectric devices inside the moons to convert tidal flexing into electricity. However, this would require gaining access to the core regions, which would be extremely difficult.
Another method would be to place satellites with powerful electromagnets in space. As they drift through Jupiter’s magnetic field, they would extract energy that could be beamed to habitats using microwave lasers. Over time, the satellites would lose momentum because of these interactions, their orbits would decay, and they would burn up in Jupiter’s atmosphere.
Ethical considerations
But as with any plans for interplanetary exploration and settlement, there is the issue of indigenous life. For Europa, scientists have long suspected that the combination of interior oceans, hydrothermal activity, and ocean floor niches could allow for life. Similar considerations have been made for Ganymede and Callisto, though Europa appears to be the most likely candidate.
If there is life within Europa’s interior oceans, any intrusions by human or robotic missions could contaminate the Europan biosphere and cause a complete collapse. This is similar to proposals for long-term human habitation and terraforming on Mars. In short, changes to the environment will inevitably threaten the destruction of local life forms.
However, one should not conclude that the presence of indigenous life means that no human habitats are possible. At the end of the day, establishing a human presence in space comes down to strategies that allow us to live sustainably away from Earth. Stringent planetary protections that prevent us from contaminating local biospheres are consistent with this goal, and might even be complementary.
In a few centuries, the human race may very well become an interplanetary species, with settlements stretching from the poles of Mercury to the Frost Line to the Kuiper Belt. While Earth will always be the cultural, spiritual, and ancestral home of humanity, the many activities that drive our civilization will be distributed throughout the Solar System.
The Jovian System, with its abundant resources, many moons, and opportunities for research, could become a major economic and scientific hub. Among the Galilean Moons, innovation incubators could be established, where researchers test and develop new methods for generating electricity from magnetic and gravitational fields.
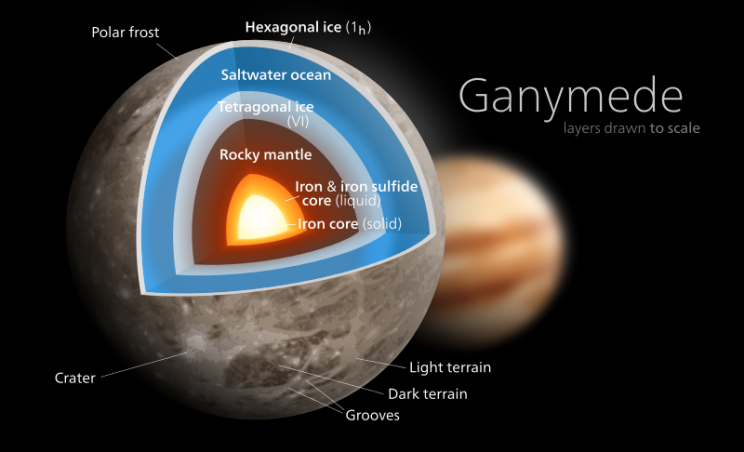
The nature of these Ocean Worlds also presents opportunities for innovative living solutions. Depending on the location, habitats could be built within surface craters or within the ice sheets. But in all cases, they will need to provide protection against the elements and have all the necessary amenities so people feel connected to the surface.
As with other destinations in the Solar System where the radiation environment is high and the local gravity is a fraction of Earth’s, the creation of long-term habitats will also enable medical research. Plants, animals, and humans in these settlements will need to undergo regular medical tests to determine the long-term effects of exposure to both.
While rotating space stations will be essential for combatting the effects of low gravity, residents could volunteer to live in low-g for years at a time as part of research experiments – similar to NASA’s Twin Study. The medical treatments derived from this could inform future missions to the edge of the Solar System and interstellar space.
As with other destinations in the Solar System, the presence of infrastructure in these systems would serve as a stepping stone. With everything from habitats and fuel depots present on (and in orbit around) the Galilean moons, missions destined to and from the outer Solar System would have a base to stop, take on supplies, rest, and refuel.
This infrastructure and the volume of traffic that would follow also mean that a thriving tourist industry would be established before long. When that day comes, people could be hearing farewell messages like this:
“From all of us here in the Jovian System, we wish you a fond farewell and hope to see you again soon! In fact, we invite you to consult our updated brochure and plan your next getaway while soaking in a detox bath of seawater and clay and readjusting to your respective gravity levels.”
“Those destined for the Cronians, Uranians, and Trans-Neptune region must remain in the outer rings for forty-eight hours before departing. Those headed for the Main Belt and inner Solar System are encouraged to complete their recovery before visiting the inner ring and its many low-g facilities.”
“We hope you enjoy the pristine views of the Galilean lights. Be sure to check out Jupiter’s aurorae and atmospheric storms while you’re at it! You’ll never be able to get this close to them again! When you reach customs, have your baggage ready for inspection. We remind you once again that the export of flora and fauna is strictly forbidden and punishable by law.”
“We hate to see you go, but we have a feeling you’ll be back. As we say in the Jovis tongue: Wit el amor hamaaree de guia, shìshì jiē possible*.”
*”With love as our guide, anything is possible.”